By Vincenzo Di Marzo
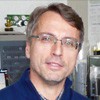
Dr. Vincenzo Di Marzo is Canada Excellence Research Chair on the Microbiome-Endocannabinoidome Axis in Metabolic Health (MEND) at Laval University, Quebec, Canada, and Research Director at the Institute of Biomolecular Chemistry of the National Research Council (ICB-CNR) in Naples, Italy. He is also the coordinator of the Endocannabinoid Research Group (www.icb.cnr.it/erg) in the Naples region, and the director of the Joint International Research Unit between the Italian National Research Council and Université Laval, for Chemical and Biomolecular Research on the Microbiome and its impact on Metabolic Health and Nutrition (MicroMeNu, www.umilaval.cnr.it). He holds a ChemD from the University of Naples in 1983, and a PhD in Biochemistry from Imperial College in London in 1988. He is co-author of over 670 articles published in peer-reviewed journals (H index 117 according to Scopus). In 2014- 2017 he has been listed among the Highly Cited Researchers (top 1% in the world) in all scientific disciplines (www.highlycited.com; http://hcr.stateofinnovation.com/). He has been the recipient of numerous research grants and awards, including: a Human Frontier Science Program research grant to study the biosynthesis, metabolism, and structure-activity relationships of anandamide; a Merkator Fellowship for Foreign Scientists by the Deutsche Forschungsgemeinshaft; the Mechoulam Award for "his outstanding contributions to cannabinoid research"; the Luigi Tartufari award for Chemistry from the Italian Academy of Sciences (Accademia dei Lincei); the "Ester Fride Award for Basic Science" from the International Association for Cannabinoid Medicines. In October 2014 he was awarded the International Award "Guido Dorso" for Research, and in May 2016 the Medal of the Italian Accademia delle Scienze, or of the XL, for Physical and Natural Sciences. In November 2018 he will be elected member of the Italian Accademia dei Lincei, the oldest science academy in the world.
The recent discussions on the possible legalization of the use of Cannabis sativa, and not only for therapeutic purposes, in several countries has revived the interest for this millenarian plant and its industrial, medicinal and recreational exploitation. Nevertheless, when speaking about cannabis, very few people remember that its true or alleged properties are due to its chemical constituents:
- its fibers (which are made of complex carbohydrates and proteins), for its use in the textile and other technological industries;
- the proteins and fatty acids (and fibers too) of its seeds, for their use in the food and possibly nutraceutical industry; and
- for cannabis flower recreational and therapeutic use, a typical class of compounds of mixed biosynthetic origin - the cannabinoids.
In fact, there are several varieties of C. sativa, with different chemical compositions that justify its different uses. As a consequence, when we talk about the possibility of separating the recreational (i.e. euphoric and mood altering) activity of cannabis preparations from their possible therapeutic activity (against a plethora of conditions, ranging from spasticity, inflammation, pain, convulsions and neurodegenerative disorders to cancer and the metabolic syndrome), in order to develop safe treatments from this plant, we need to understand how such preparations differ in their compositions in cannabinoids that exert psychotropic and therapeutic effects (i.e. Δ9-tetrahydrocannabinol [THC]), or only the latter (e.g. cannabidiol [CBD]). Furthermore, since all plant cannabinoids are present in cannabis flowers as carboxylic acid precursors, from which they are produced following desiccation- or heating-induced decarboxylation, even for a given variety of cannabis the type of cannabinoids that it contains depends on flower storage and treatment. Finally, cannabinoids are lipophilic compounds (they do not dissolve easily in water), and therefore any preparation of cannabis flowers to be used for therapeutic purposes must take into account that administration routes such as oral infusions may not deliver amounts of these compounds that are sufficient to produce the desired effect.
This long preamble is to state that the first thing to remember about cannabis is that there is no such a thing like "one cannabis", and therefore when someone asks: "What do you think of the therapeutic use of cannabis?" the correct answer is, unfortunately, another series of questions: "Which one? Stored and administered how?". In fact, I believe that it is much better to talk about the potential therapeutic actions of the cannabinoids, much in the same way also the layman now knows that the nutritional properties of cannabis seeds, like other foods, are due to their peculiar proteins, fibers and fatty acids. What do we know about cannabinoids? We know a lot about THC, whose pharmacological effects on mammals and humans are almost uniquely due to its capability of activating two G-protein-coupled receptors (GPCRs) on the cell external membrane, the cannabinoid receptor type-1 (CB1) and type-2 (CB2) (1). The euphoric properties of THC are due to the former of these two receptors, and therefore when the CB2 receptor were discovered everybody immediately thought that it could be targeted selectively with synthetic ligands, thus producing a series of therapeutic effects (against, among others, several inflammatory and auto-immune disorders, pain, fibrosis and osteoporosis) without the unwanted psychotropic effect of THC (2). Indeed, a lot of activity is ongoing to develop such compounds for clinical evaluation, and as possible replacements for THC (marinol, dronabinol) and its derivatives (e.g. nabilone). Since it was clear that not only defective activity of CB1, but also overactivity of this receptor may underlie disease in animal models (for example, obesity, hyperphagia and substance of abuse reinstatement, schizophrenia, metabolic disorders, and liver, kidney and lung fibrosis) (3,4), synthetic compounds antagonising the action of CB1 were developed and found to be effective in clinical trials against obesity and type 2 diabetes (4). Unfortunately, however, since, unlike CB2, CB1 is very abundant in neurons of the mammalian brain, and is now known to be very important to counter the consequences of stress, chronic reduction of CB1 activity with CB1 antagonists was also accompanied by signs of anxiety, depression and suicidal ideation in some obese patients, and the clinical development of these compounds had to be abruptly interrupted (4).
The presence of CB1 and CB2 in mammals pointed to the existence of endogenous ligands for these receptors. These metabolites, namely anandamide and 2-arachidonoylglycerol (2-AG), were discovered in the 1990's (5,6), and soon named endocannabinoids (7). Enzymes responsible for their biosynthesis and inactivation were identified and characterised between the end of last century and the beginning of the present one (8-11). These discoveries, and the realization that endocannabinoid tissue concentrations are altered in animal models of most disorders during either physiological (night-light cycles, short term food deprivation and re-feeding, estrous cycle, short term stressful stimuli at the cellular and organism level, etc.) or pathological alterations of the cell homeostasis, led to the suggestion that the endocannabinoid system – i.e. the ensemble of endocannabinoids, their metabolic enzymes, and CB1 and CB2 receptors – is activated "on demand" in a cell- and time-specific manner to attempt restoring homeostasis. Additionally, it became clear that, exactly like other endogenous pro-homeostatic signaling systems (i.e. the immune system), the endocannabinoid system can become deranged during prolonged perturbations of the cell and organ steady-state, and hence occasionally contribute to disease symptoms or progress. These hypotheses have been increasingly confirmed over the last two decades, thus leading to hypothesize that inhibitors of endocannabinoid biosynthesis or inactivation could replace CB1 agonists (e.g. THC and its synthetic analogs) or antagonists, respectively, as more specific, and hence safer, therapeutic drugs, since they would only act "when and where" endocannabinoids are produced to play a protective or counterprotective action (12). As a consequence, over the last 15 years much effort has been made to develop clinically safe inhibitors of anandamide and 2-AG production or degradation (12). This strategy, however, has been complicated by the fact that the biosynthetic and catabolic pathways of endocannabinoids are redundant and shared with other bioactive lipid mediators that do not act on cannabinoid receptors but have molecular targets with actions often opposite to CB1 and CB2. In fact, we now know that probably hundreds of such endocannabinoid-like mediators exist, and constitute a true "extended endocannabinoid system" or endocannabinoidome (1). Thus, inhibiting endocannabinoid inactivation, for example, will lead to enhance the levels also of endocannabinoid-like molecules, and, subsequently, modulate the activity of the targets for these molecules. This, however, should not discourage drug developers from attempting to target the endocannabinoid system for therapeutic use, since it is possible, for example, to develop, in a rational manner, multi-target inhibitors of endocannabinoid biosynthetic or degrading enzymes that at the same time block the effect of endocannabinoid-like mediators at other targets (1).
The recent difficulties of developing synthetic endocannabinoid-based drugs coincided with renewed enthusiasm towards exploiting the potential therapeutic effects of non-psychotropic plant cannabinoids. In fact, and funnily enough, of about 100 such compounds found to date in several varieties of C. sativa, only THC is capable of activating CB1 (and CB2) receptors - which, incidentally, in my opinion should be renamed "THC receptors" instead of "cannabinoid receptors". This also in consideration of the fact that other cannabinoids have not been found so far to act only on one type of specific receptor, and appear instead to be modulating - not necessarily with high potency - the activity of either previously known or "new" orphan receptors. In particular, CBD has been the object of a plethora of pharmacological investigations that led to identify for this compound a dozen of potential molecular targets, some of which (like some thermo-sensitive transient receptor potential [TRP] channels, peroxisome proliferator-activated receptors [PPARs], and orphan GPCRs) are also receptors for endocannabinoid-like mediators, and therefore belong to the endocannabinoidome. Furthermore, studies in experimental models of, among others, (neuro)inflammatory, neurological, skeletal muscle, oncological and metabolic disorders, suggested that CBD, acting simultaneously at some of these targets, could be a valuable therapeutic tool. It was also hypothesised that CBD, again thanks to its multi-target nature, could "tame" some of the unwanted central effects of THC, thus widening its narrow therapeutic window (i.e. the difference between the most effective and the highest safe doses).
This led to the development of Sativex, a combination of two botanical extracts from two different varieties of C. sativa, one rich in THC and the other in CBD, and delivering, through an oromucosal spray, an approximate 1:1 ratio of the two cannabinoids. This first-in-its-class drug is currently marketed in over 30 countries against spasticity and (only in Canada) neuropathic pain in multiple sclerosis patients (13). More recently, the therapeutic potential of botanical CBD per se against seizures in rare and untreatable forms of pediatric epilepsy was also recognised in several clinical trials, and the compound was subsequently approved by the FDA for this indication as Epidiolex (14). The results of other clinical trials with Sativex (in oncological chronic pain, glioblastoma and Huntington's disease), CBD (for example in dyslipidemic type 2 diabetes and schizophrenia) and Δ9-tetrahydrocannabivarin (THCV) (in type 2 diabetes) have been reported, with mixed, but nevertheless, always promising results and untroubled safety profiles.
In summary, with the discovery of the endocannabinoid system first, and the endocannabinoidome later, and the "rediscovery" and clinical development of botanical cannabinoids, the long way to the rational design of new therapies from C. sativa has finally reached its first major milestone. I believe that, in consideration of their several potential clinical implications, the successes obtained so far (despite the many complications of, and challenges imposed by, the complex biochemistry and physiology of endocannabinoids and the pharmacology of non-psychotropic plant cannabinoids [15]), rather than the disappointments, should really count when deciding whether or not we should make further efforts to enlarge and improve the therapeutic armamentarium generated from the study of cannabis and its components.
Bibliography
1. Di Marzo V. "Nuevos enfoques y retos de la orientación en el sistema endocannabinoide". Nat Rev Drug Discov. (2018) [Epub ahead of print] Review.
2. Morales, P. et al. Cannabinoid receptor 2 (CB2) agonists and antagonists: a patent update. Expert Opin. Ther. Pat. 26, 843–856 (2016).
3. Roser, P., Vollenweider, F. X. & Kawohl, W. Potential antipsychotic properties of central cannabinoid (CB1) receptor antagonists. World J. Biol. Psychiatry 11, 208–219 (2010).
4. Tam, J. et al. The therapeutic potential of targeting the peripheral endocannabinoid/CB1 receptor system. Eur. J. Intern. Med. 49, 23–29 (2018).
5. Devane, W. A. et al. Isolation and structure of a brain constituent that binds to the cannabinoid receptor. Science 258, 1946–1949 (1992).
6. Mechoulam, R. et al. Identification of an endogenous 2‑monoglyceride, present in canine gut, that binds to cannabinoid receptors. Biochem. Pharmacol. 50, 83–90 (1995).
7. Di Marzo, V. & Fontana, A. Anandamide, an endogenous cannabinomimetic eicosanoid: 'killing two birds with one stone'. Prostaglandins Leukot. Essent. Fatty Acids 53, 1–11 (1995).
8. Okamoto, Y. et al. Molecular characterization of a phospholipase D generating anandamide and its congeners. J. Biol. Chem. 279, 5298–5305 (2004).
9. Bisogno, T. et al. Cloning of the first sn1‑DAG lipases points to the spatial and temporal regulation of endocannabinoid signaling in the brain. J. Cell Biol. 163, 463–468 (2003).
10. Cravatt, B. F. et al. Molecular characterization of an enzyme that degrades neuromodulatory fatty-acid amides. Nature 384, 83–87 (1996).
11. Dinh, T. P. et al. Brain monoglyceride lipase participating in endocannabinoid inactivation. Proc. Natl Acad. Sci. USA 99, 10819–10824 (2002).
12. Di Marzo, V. Targeting the endocannabinoid system: to enhance or reduce? Nat. Rev. Drug Discov. 7, 438–455 (2008).
13. Keating, G. M. Δ9-Tetrahydrocannabinol/Cannabidiol oromucosal spray (Sativex): a review in multiple sclerosis-related spasticity. Drugs 77, 563–574 (2017).
14. Devinsky, O. et al. Trial of cannabidiol for drug resistant seizures in the Dravet syndrome. N. Engl. J. Med. 376, 2011–2020 (2017).
15. Ligresti, A., De Petrocellis, L. & Di Marzo, V. From Phytocannabinoids to Cannabinoid Receptors and Endocannabinoids: Pleiotropic Physiological and Pathological Roles Through Complex Pharmacology. Physiol. Rev. 96, 1593–1659 (2016).